Tackling Antibiotic-Resistant Staphylococcus aureus with Phagemids and Cas13a
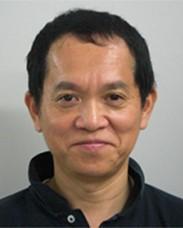
Bacteriophage therapy has long been heralded as one of the most promising avenues in the treatment of antibiotic-resistant bacterial infections. In many ways, using bacteriophage (or simply, phage) is a no-brainer: the most abundant microbes on the planet, phage exhibit high host specificity and cannot infect animal cells, allowing for the destruction of targeted bacteria without affecting the rest of the microbiome.
In the CRISPR era, phage can now be engineered to be even better killing machines. Companies like SNIPR Biome, Locus Biosciences, and Eligo Bioscience have all developed CRISPR-armed phage, either to kill specific pathogenic bacteria or (in the case of Eligo) edit the bacterial genome in vivo. Yet the road to clinical translation has been riddled with failures and to date, no phage therapy has been approved for use in humans.
Longzhu Cui, a Professor at Jichi Medical University in Japan, has spent the last decade of his career trying to change this. In a recent paper published in Communications Biology, Cui and his team presented a novel method that he believes may help overcome some of the previous limitations of phage therapy.
Using phagemids as a packaging system, the team created non-replicative phage that deliver CRISPR-Cas13a system to selectively eliminate methicillin-resistant Staphylococcus aureus (MRSA). »This method was chosen primarily to support the development of biocontainment-enhanced, phage-based antimicrobial agents, which help reduce the biosafety risks associated with potential adverse effects of wild-type phage,« Cui explained.
Bacteriophage as drug delivery systems: the phagemid approach
Originally from China, Cui spent the early part of his career as a medical doctor and clinician, working in the field of infectious disease. After a short stint in South Korea, Cui moved to Japan, where he has spent the last two decades researching drug resistance mechanisms in bacteria, and phage-based drug development.
He said that his decision to pursue phage therapy was born of a desire to return positive results and new therapies to the patient community, particularly those suffering from drug-resistant bacterial infections.
Cui’s lab of 34 researchers has previously taken a different approach to MRSA phage therapy, using a mobile genetic element called S. aureus pathogenicity island (SaPI) to package CRISPR-Cas13a into phage. However, SaPI’s virulence potential and the associated risk of horizontal gene transfer is likely to hinder its clinical applications. In the current paper, the team instead used phagemids as a packaging vector.
What are phagemids?
As the name suggests, phagemids are, essentially, hybrids between phage and plasmids. They can be used as packaging vectors for genetic cargo in molecular biology. For example, plasmids can be engineered to contain a portion of DNA from the filamentous M13 bacteriophage that codes for a packaging signal. This allows the resulting phagemids to be recognised by the phage packaging machinery and packaged into the M13 phage capsids. Consequently, the genetic cargo they carry can be delivered to the phage host bacteria.
The team’s reasoning behind using phagemids was threefold. First, phagemids are easy to genetically modify, particularly when compared to engineering phage themselves. Second, their use eliminates the risk of unwanted horizontal gene transfer. Finally, the encapsulation of the phagemids results in non-replicative phage particles, minimising other safety risks associated with replicative, wild-type phage.
Cas13a-armed phage allow targeted bacterial killing in vitro
The team created phagemids containing packaging signals for a phage with a broad host range called Tan2, as well as the sequences for CRISPR-Cas13a and a gRNA targeting one of S. aureus’ methicillin resistance genes mecA. The phagemids were encapsulated by the Tan2 phage, creating antimicrobial capsids (AB-capsids).
Several other approaches to CRISPR-engineered phage therapy, including those explored by Locus Biosciences and SNIPR Biome, use Cas3, a CRISPR system that shreds large sections of DNA after target recognition, resulting in the death of the bacterial cell. This is preferable to, for example, targeting with traditional CRISPR-Cas9, which creates a double-stranded break that can sometimes be repaired by the bacteria.
Cui remarked that a similar killing effect can be achieved using CRISPR-Cas13a (C2c2), an RNA-targeting nuclease. Cas13a is notorious for its collateral activity: once it recognises its target mRNA transcript and cleaves it, it also destroys all the other transcripts within the cell, typically resulting in cell death. This collateral activity makes it unappealing for use in humans but attractive for the targeted destruction of pathogenic bacteria.
After optimising packaging efficiency and minimising contamination with wild-type phage, Cui and his team tested their AB-capsids on S. aureus in vitro to assess their bactericidal efficacy. The capsids targeting the mecA gene successfully killed MRSA without affecting another non-resistant strain, demonstrating their sequence-specific killing capacity.
After the success of these initial experiments targeting mecA, they subsequently adapted the system to target various other S. aureus antibiotic resistance genes to ensure the system would be applicable across a broad spectrum of resistant strains. These studies confirmed the ability of the system to selectively kill clinical isolates of resistant S. aureus in an in vitro setting (see Figure 1 below).
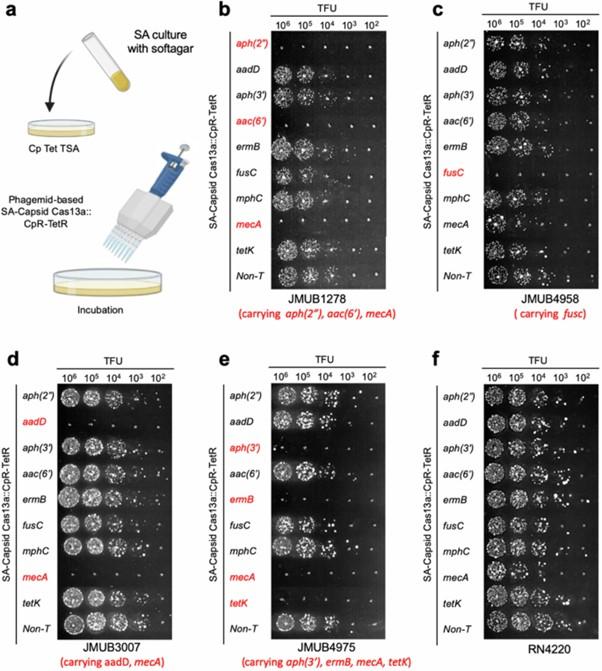
Optimisation for future in vivo studies
While the AB-capsids allowed for targeting of MRSA, their killing capacity was not as strong as wild-type phage, largely owing to their inability to replicate and infect more bacterial cells. Cui noted that improving killing efficiency is the focus of ongoing research. The team is also pursuing several other approaches to optimise the system, including modifying Cas13a to enhance its killing efficiency and reduce its size, and tweaking the gRNA design in order to target the most vulnerable mRNA regions.
Another limitation of the study is the contamination by wild-type phage, which the team noted in the paper as preventing them from forming a clear picture of the efficacy of the AB-capsids. They also remarked that comprehensive analysis of the off-target effects of the system is also needed to determine its potential for clinical translation.
In terms of in vivo studies, disease modelling is a challenge; adult mice are not a natural host of S. aureus, so they require very high doses to manifest infection symptoms. To address this, Dr. Cui’s team has used newborn mice, whose immature immune systems allow for infection at lower bacterial doses, closer to levels observed in human infections. While the data remains unpublished, Cui said the results so far have been promising.
Cui hypothesised that the lack of success of phage therapy can be attributed to patient concerns regarding the safety of using living materials like viruses as medicine and to a lack of investment by pharmaceutical companies who know all too well the difficulties of patenting phage therapies and the associated regulatory hurdles.
Nonetheless, Cui said that he remains hopeful that with further research, the study will have a positive impact: »If this approach demonstrates clear advantages in efficacy and specificity over traditional antibiotics, particularly against drug-resistant MRSA, it could gradually gain acceptance, driven by the urgent need for innovative treatments in the face of rising antibiotic resistance.«
Rebecca Roberts is a molecular biologist, science writer/communicator, and scientific marketing professional based in Queensland, Australia.
To get more CRISPR Medicine News delivered to your inbox, sign up to the free weekly CMN Newsletter here.
Tags
CLINICAL TRIALS
Sponsors:
Suzhou Maximum Bio-tech Co., Ltd.
Sponsors:
Zhejiang University