Circular Single-Stranded DNA Enables Efficient Non-Viral Immune Cell Engineering: Implications for CAR-T Cell Therapy
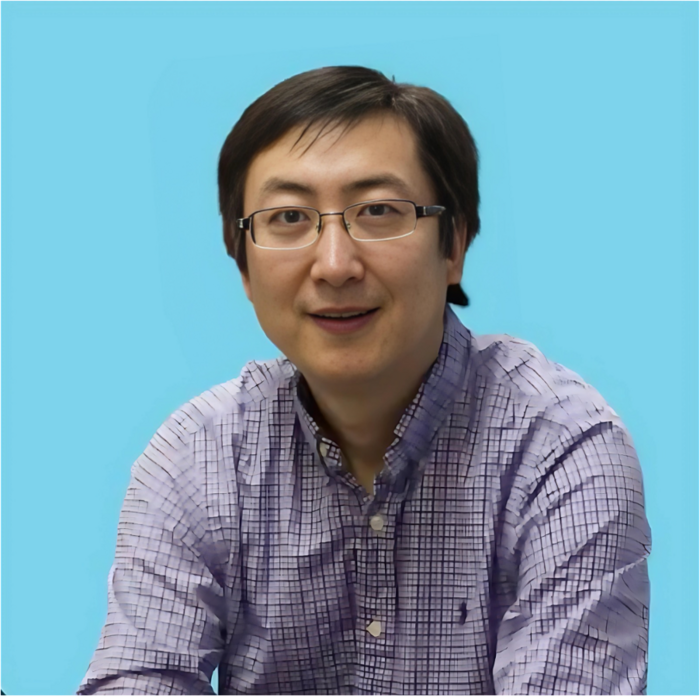
In a recent study, scientists at Full Circles Therapeutics developed a novel DNA template system to engineer immune cells for therapeutic purposes. The researchers demonstrated that their novel mini-circular single-stranded DNA (cssDNA) technology, termed GATALYST, enables more efficient and safer genetic modifications in various cell types, including induced pluripotent stem cells (iPSCs) and immune cells used in cancer immunotherapy. The system achieved up to 70% gene integration in some cell types while maintaining high cell survival rates.
»When we use conventional double-stranded DNA as a donor, we typically achieve only single-digit percentage integration rates. However, with cssDNA, we can achieve much higher efficiency, with integration rates of 50%–70%,« said Howard Wu, PhD, co-founder and CSO at Full Circles Therapeutics, and corresponding author of the study. »This higher efficiency saves significant time and resources, potentially reducing the cost of therapeutic products. Ultimately, this makes the treatment more affordable and accessible to patients.«
The study was published in Nature Biotechnology.
Unmet need: Efficient delivery of therapeutic transgenes for cell engineering
Although CRISPR/Cas9 has enabled targeted gene ablation with high efficiency, precise transgene integration remains a challenge. Delivering gene-editing components using viral particles, such as adeno-associated virus (AAV) vectors, faces several limitations, including safety concerns, manufacturing constraints, and limited payload capacity.
»The field has long sought effective non-viral gene-editing technologies,« noted Dr. Wu. »Accumulating data have revealed potential safety concerns with viral vectors, including adverse effects in AAV trials, some of which have resulted in patient deaths.«
Non-viral approaches using double-stranded DNA (dsDNA) and linear single-stranded DNA (lssDNA) have also been explored, but these methods suffer from low efficiency, high cytotoxicity, and scalability issues. These limitations pose challenges for the production of engineered immune cells for cancer therapy, such as chimeric antigen receptor (CAR)-T cells.
Genome writing catalyst: A novel method for precise transgene integration
To address the limitations of current methods for targeted gene insertion, researchers developed a novel cssDNA-based approach for precise genomic integration. The genome writing catalyst system, termed GATALYST, leverages cssDNA as donor templates for homology-directed repair (HDR)-mediated genome editing.
Dr. Wu described the three-component system as containing a guide RNA to target the editing machinery onto a specific location in the genome, a nuclease, and the system’s key innovation, a cssDNA molecule as a donor.
The research team employed a proprietary M13 phagemid system to produce ultrapure cssDNA up to 20 kb in length, which is more than four times the capacity of viral vectors. The system involves co-transformation of E. coli with a recombinant phagemid and a helper plasmid, resulting in the secretion of phage particles containing cssDNA into the culture medium (Figure 1). The cssDNA is then purified using anion exchange chromatography, achieving over 95% purity across various lengths.
Dr. Wu emphasised that the system is highly flexible, as the phagemid vector can be adapted to contain the desired genetic sequence. He added that the cssDNA manufactured from this process does not entail antibiotic resistance genes or other bacterial elements.
The circular structure made the resulting cssDNA molecules very stable, maintaining their integrity after multiple freeze-thaw cycles (up to 42 times over 14 days). Moreover, the cssDNA molecules were more resistant to exonuclease T-mediated degradation than linear DNA molecules.
Although cssDNA molecules were stable, their half-life was considerably shorter than that of dsDNA (5.8 hours for cssDNA vs. 42 hours for dsDNA) in dividing cells. Dr. Wu explained that the shorter half-life of cssDNA in cells is an advantage, as it could result in reduced cellular toxicity and lower risk of off-target recombination, which is commonly seen in dsDNA-mediated gene integration.
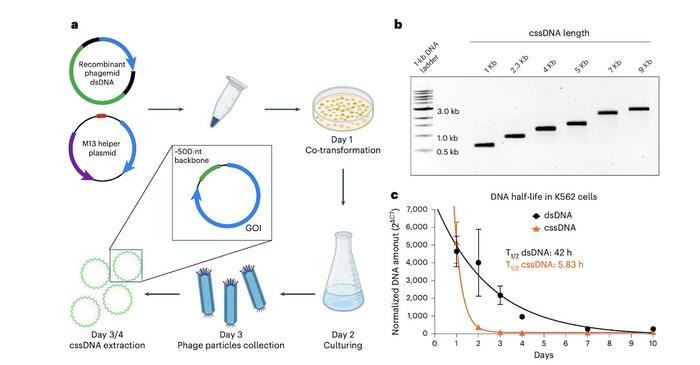
GATALYST provides high integration efficiency in multiple cell types
To evaluate the efficiency of cssDNA-mediated genome editing, the team conducted experiments in multiple cell types, including K562 cells (human leukaemia cell line), induced pluripotent stem cells (iPSCs), primary T cells, natural killer (NK) cells, B cells, and haematopoietic stem cells. They compared the knock-in efficiency and cell viability of cssDNA with dsDNA and lssDNA donor templates, using GFP reporter genes and functional CAR constructs as payloads.
The cssDNA donor templates demonstrated superior integration efficiency compared to dsDNA and lssDNA across multiple cell types. In K562 cells, cssDNA achieved over 45% knock-in efficiency with 3 μg of donor template while maintaining high cell viability (>80%). In contrast, dsDNA showed low efficiency (<10%) and severe cytotoxicity (<10% viability at 3 μg) (Figure 2).
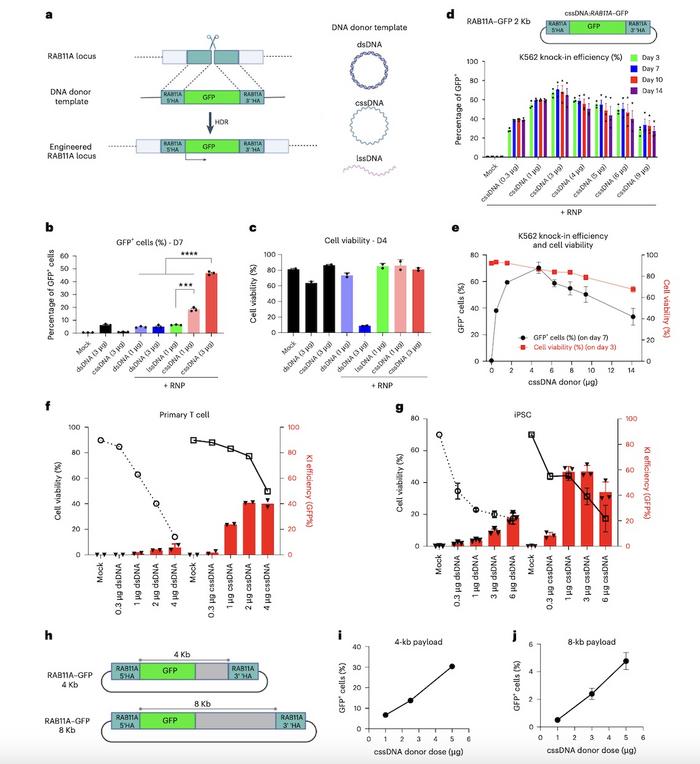
In iPSCs, cssDNA targeting the RAB11A locus achieved approximately 50% knock-in efficiency without compromising cell health, outperforming dsDNA (~5% knock-in efficiency). The highest knock-in efficiency in iPSCs was close to 70% with 3 μg of RAB11A-targeting cssDNA, and similar results were observed for the B2M locus. When using two fluorescent reporter cssDNAs (GFP and mCherry), bi-allelic integration at RAB11A was achieved, with ~15% of cells expressing both reporters.
In primary T cells, cssDNA achieved high knock-in efficiency at both the RAB11A and TRAC loci, with higher efficiency observed for smaller payloads (2 kb vs 4 kb). The addition of the DNA-PK inhibitor M-3814 further increased the knock-in efficiency by 60%–150%.
The knock-in efficiency of cssDNA templates was also higher than that of dsDNA templates in other primary immune cells, including NK cells (40% vs 7%), B cells (24% vs 15%), and CD34+ haematopoietic stem cells (20% vs 6%) (Figure 3). Dr. Wu emphasised that, unlike viral vectors that exhibit limited tropism, this method is tissue-agnostic, as illustrated by the wide range of clinically relevant cell types that can be engineered using this method.
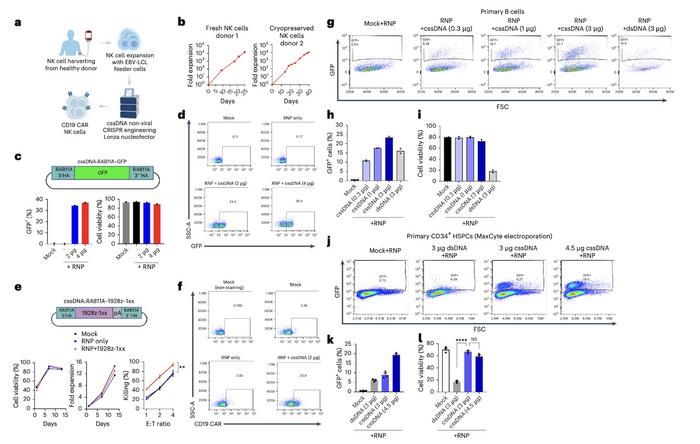
GATALYST provides improved safety
The study demonstrated that cssDNA exhibited superior safety profiles compared to dsDNA across multiple cell types. In K562 cells, cssDNA maintained over 80% cell viability even at high doses of up to 5 μg. In contrast, cells exposed to 3 μg of dsDNA showed 10% cell viability.
In primary T cells, cssDNA did not trigger significant cellular proinflammatory responses, unlike dsDNA, which induced high levels of IFN-γ and TNF-α and activated numerous innate immune response genes. RNA sequencing analysis confirmed that although dsDNA highly activated intracellular IFN-γ response genes, cssDNA treatment showed no significant changes in these inflammatory pathways.
Similarly, in iPSCs, cssDNA maintained high cell viability and did not compromise cell health or pluripotency, as demonstrated by normal karyotype analysis, and maintained the expression of self-renewal genes. In primary NK cells, B cells, and CD34+ haematopoietic stem cells, cssDNA consistently showed better cell viability than dsDNA.
Dr. Wu attributed the enhanced safety profile of cssDNA partly to its shorter half-life: »The cssDNA has advantages owing to its short half-life in the cytoplasm and its enhanced ability to enter the nucleus. This structure helps bypass cellular DNA surveillance mechanisms and evade immunogenic pathways.«
Functional CAR-T cell engineering
According to Dr. Wu, the most immediate impact of this technology is the production of CAR-T cells for cancer therapy. Using cssDNA, the team achieved ~55% knock-in efficiency for a CD19/CD22 bispecific CAR at the TRAC locus in primary T cells. These engineered CAR-T cells demonstrated potent and durable anti-tumour efficacy both ex vivo and in vivo (Figure 4). The high efficiency of the system could make CAR-T cell production more reliable and cost-effective, potentially improving access to life-saving therapies.
»Previous approaches using different modalities achieved only 10%–20% knock-in efficiency for CAR molecules. Our method achieves approximately 50% CAR knock-in efficiency, which could be further improved through process optimisation,« Dr. Wu noted. »This advancement enables us to produce more efficiently engineered CAR-T cell therapies for treating autoimmune disorders and blood cancers, making the treatment more affordable and accessible.«
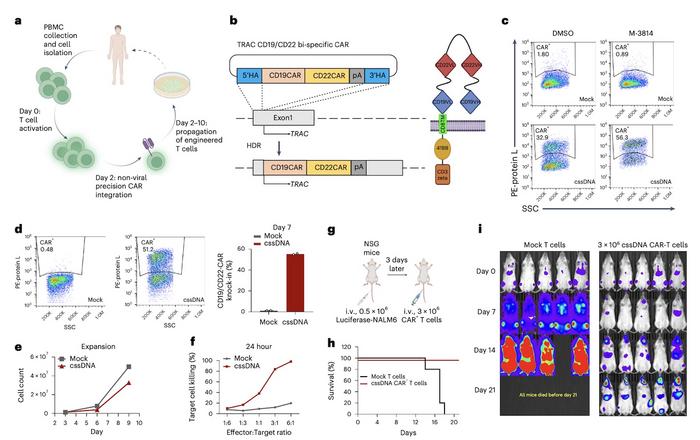
Potential challenges and future directions
Dr. Wu acknowledged that scaling up cssDNA production for commercial use presents challenges. To address these challenges, the team is developing an approach that uses bacterial fermentation. »We engineered the phage-derived particles to remove unnecessary sequences from the circular DNA,« Dr. Wu explained. »The current challenge of scaling up manufacturing is essentially a matter of fermentation optimisation.«
Bacterial fermentation is commonly used for the production of DNA and recombinant proteins, including cssDNA, which is a mixture of both. »To scale up cssDNA manufacturing, we are optimising the upstream process to meet GMP-grade standards for production at gram scales,« said Dr. Wu. »As with other fermentation-based biologics, endotoxin removal is critical before clinical use. We have developed a chromatography-based downstream process that effectively reduces endotoxin levels to meet regulatory requirements.«
A key differentiator of GATALYST cssDNA is that it is free from antibiotic resistance genes and bacterial cis-regulatory elements, which can complicate regulatory compliance. »Our engineered phagemid system, which splits the f1 bacterial origin, ensures that redundant sequences are looped out in a precise way during cssDNA synthesis and phage assembly in the host bacteria, resulting in a compact, regulatory-compliant product,« Dr. Wu explained: »This approach ensures that we can seamlessly adopt a traditional upstream fermentation process for the production of a mini-version of cssDNA product.«
Another challenge is the delivery of cssDNA for efficient in vivo gene editing, a goal that many researchers are working to achieve. »We recently demonstrated successful liver delivery without triggering significant immune responses, enabling targeted genome integration in follow-up studies. Additionally, promising results have been observed in inner ear cochlear delivery,« Dr. Wu said. »We are optimistic that these exciting findings will pave the way for the next chapter in cell and gene therapy, particularly for non-viral in vivo gene integration-based root-cause therapies.«
Dr. Wu also emphasised that the versatility of the system and its ability to handle larger genetic sequences opens new possibilities for cell engineering, potentially enabling the development of effective cellular therapies for multiple diseases. »Integrating large payloads is crucial for addressing challenges that current viral approaches cannot solve,« he said. »GATALYST can accommodate sequences of up to 20 kb, opening up new therapeutic possibilities for conditions such as cystic fibrosis, haemophilia A, and hereditary hearing loss, where the disease-causing genes are too large for current viral vectors to handle.«
Dr. Wu sees this technology as part of a broader evolution in therapeutic approaches: »As we mark the 70th anniversary of the discovery of the DNA double helix, we have seen remarkable developments in therapeutic approaches from antibody-based drugs to mRNA vaccines. I believe the next frontier involves working directly with DNA by challenging the central dogma to establish a new standard for genetic medicine. We hope this emerging modality will soon benefit patients suffering from various diseases.«
Link to the original article in Nature Biotechnology:
Christos Evangelou, PhD, is a freelance medical writer and science communications consultant.
Tags
CLINICAL TRIALS
Sponsors:
Primary sponsor: The First Affiliated Hospital of Bengbu Medical College Primary sponsor's address: 287 Changhuai Road, Longzihu District, Bengbu, Anhui, China Secondary sponsor: Country: China Province: Anhui City: Bengbu Institution hospital: The First Affiliated Hospital of Bengbu Medical College Address: 287 Changhuai Road, Longzihu District