Gene Editing for Muscular Dystrophies: Where Do We Stand?
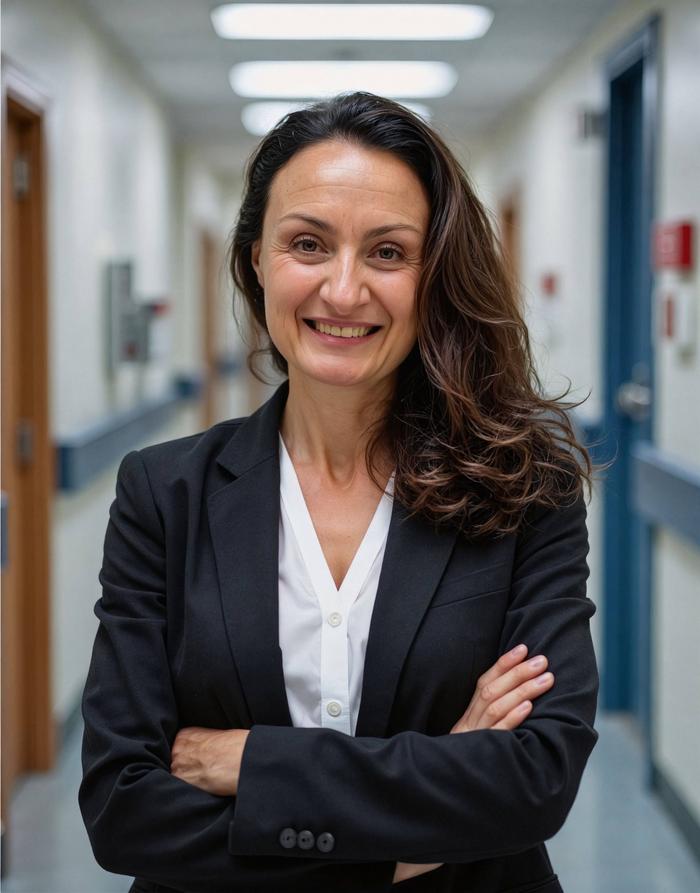
The identification of the DMD gene in the late 1980s marked a milestone in modern human genetics. Since then, research into Duchenne muscular dystrophy has spearheaded research into other muscle conditions as well as into advanced therapies and gene editing for rare diseases.
As a researcher specialising in neuromuscular diseases, I outline through selected examples how gene editing is being harnessed to treat various muscular dystrophies. I also highlight how each application is suited to their particular genetics and note common challenges. To narrow down this extensive field of research, I focus on strategies that are at least at the advanced preclinical stage of research in animal models or have advanced into clinical development.
What are muscular dystrophies?
Muscular dystrophies are a group of neuromuscular disorders where the primary defect lies in the muscle fibre, its surrounding basal lamina or the adjacent extracellular matrix. Some muscular dystrophies may also affect cardiac muscle and the nervous system.
They are extremely heterogeneous in their genetic origin and how the condition presents clinically; they can be X-linked or autosomal, recessive or dominant or both, or caused by expansion or contraction of repeat sequences. Potentially, they can all be corrected by the growing array of gene-editing technologies.
Duchenne muscular dystrophy
Duchenne muscular dystrophy (DMD) is the paradigm of an inherited dystrophic muscle disease. DMD was mapped to the X chromosome and the DMD gene was identified in the late 1980s. Becker muscular dystrophy (BMD) is an allelic milder form of the disease. DMD is one of the most thoroughly investigated muscular dystrophies for which there are very well characterised mouse models as well as canine and other larger animal models.
Induced pluripotent stem cell (iPSC) technology has been exploited to generate physiological skeletal or cardiac muscle cell models for DMD representing many different human mutations. Knowledge gained through those efforts ultimately led to DMD becoming the first muscular dystrophy, and one of the first human monogenic diseases, to profit from the newly-born CRISPR/Cas9 gene-editing technology more than 10 years ago.
Since then, researchers have found that DMD and its models provide a rich platform for exploring the expanding gene-editing toolbox's possibilities, whether correcting insertions or deletions, addressing point mutations, or modifying transcription of the DMD gene or other compensatory genes.
Approved genetic medicines for Duchenne muscular dystrophy
DMD is also at the forefront of genetic therapies, including those based on gene addition, exon skipping, and gene editing.
While the AAV-mediated microdystrophin gene therapy Elevidys (developed by Sarepta Therapeutics and Roche) is the only approved gene addition therapy for DMD, others are in the pipeline. Microdystrophin contains the regions of full-length dystrophin that are considered critical for its function, namely the N-terminus and truncated central-rod and C-terminus domains. It is produced from a shortened microdystrophin transgene (approximately 4 kb long in contrast to the more than 11 kb full cDNA) designed to fit within an AAV vector (reviewed in 1).
Beyond gene therapy, several splice-switching antisense oligonucleotides are approved in the US and other non-European countries. These work by inducing the skipping of certain exons of the DMD gene so that the reading frame is restored, leading to the translation of a shorter but partially functional dystrophin protein. These include eteplirsen, golodirsen and casimersen (developed by Sarepta Therapeutics) and vitolarsen (developed by NS Pharma).
While these therapies may slow disease progression, the impact on dystrophin re-expression and clinical improvement is modest, and they must be administered every few weeks. This huge burden for patients and families calls for more efficient and longer-lasting alternatives such as therapies based on gene editing, which offer the potential for durable treatment with minimal interventions.
Clinical-stage gene-editing therapies for Duchenne muscular dystrophy
Gene therapy and gene editing are distinct approaches each with their pros and cons. In broad terms, editing of the DNA allows precise and permanent correction of the specific genetic defect. In contrast, gene therapy (understood as providing a full or truncated form of the defective or missing gene) relies on the long-term expression of an exogenous transgene. Furthermore, and as exemplified below, with gene editing it is possible to exploit the cell's own regulatory mechanisms to modulate (upwards or downwards) the expression of genes of interest.
A search in clinicaltrials.gov (as of the 7th of May 2025) using the term 'CRISPR' retrieves 122 studies, 39 of which are listed as 'recruiting'. When the term 'Muscular Dystrophy' was added to the search, three studies were retrieved.
One of these (NCT06594094, MUSCLE) is sponsored by HuidaGene Therapeutics, which is advancing HG302 as a gene-editing therapy for DMD. In that trial, HG302 is being evaluated in children between 4 and 8 years old. HG302 is developed using a proprietary version of Cas12Max, a high fidelity and compact size type V Cas protein, targeting the human DMD exon 51 splice donor site through a specific gRNA. In December 2023, HuidaGene reported data from a humanised mouse model showing that a single injection of HG302 led to stable genome editing with minimal off-target effects, functional dystrophin expression and muscle function improvement, paving the way for investigation in humans.
The MUSCLE trial is assessing the safety and durability of a single intravenous injection of an AAV vector to deliver HG302. At the CRISPR Medicine Conference 2025 held last month in Copenhagen, HuidaGene Therapeutics' CEO, Dr. Alvin Luk, disclosed preliminary clinical data three months after the first two patients were injected with a low dose of HG302. The data emphasised the safety of HG302 administration with no severe adverse events, including thrombotic microangiopathy, as well as positive changes in the first treated patient in two of the common motor functional outcome measures: the 6 minutes walking test (6MWT) and the North Star Ambulatory Assessment (NSAA).
Another registered clinical trial (NCT06392724) sponsored by Peking Union Medical College Hospital) is evaluating the therapeutic potential of a dual AAV9 vector to deliver a base editor to skip exon 50 of the DMD gene in children aged 4 to 10 years old. Importantly, this study addresses a major concern of systemic viral gene delivery, which is pre-existing immunogenicity to AAV antigens. The trial will include a comprehensive short-term prophylactic immunosuppression regimen (with rituximab and sirolimus) to mitigate potentially harmful immune responses to the therapeutic candidate, GEN6050X. This safety measure is particularly critical in light of a case where a young adult with DMD died approximately one week after receiving an AAV gene therapy designed to drive dystrophin upregulation using a catalytically-inactive Cas9 bound to a transcriptional activator (VP64 domain). Despite receiving rituximab and sirolimus, the patient experienced cardiac and pulmonary failure, with post-mortem studies indicating that immunity to the AAV capsid itself, rather than to the gene-editing machinery, was the likely cause of death (2).
Beyond DMD: Gene editing for limb-girdle muscular dystrophies (LGMD)
Clinical progress
Gene-editing approaches to treat muscular dystrophies beyond DMD are advancing rapidly, with the first clinical studies in the pipeline. One notable example is the limb-girdle muscular dystrophies (LGMD), a group of over 30 distinct genetic entities with a dominant or recessive mode of inheritance.
A search of the clinicaltrials.gov database for muscular dystrophies other than DMD retrieves a Phase 1/2a study (NCT05588401, status: ‘not yet recruiting’) led by Prof. Simone Spuler at Charité University in Berlin. This trial will evaluate the safety and efficacy of autologous gene-edited muscle stem cells (known as GenPHSats-bASKet) in individuals with LGMD who are older than 14 years old. MyoPax, the company behind GenPHSats, reports on its website that a first-in-human trial is at an advanced stage of planning.
Ex vivo approaches for LGMD2B
Pre-clinical research on LGMD2B by the group of Prof. Spuler was published by Dr. Escobar Fernandez in January 2025 (3). LGMD2B is a recessive form of LGMD caused by biallelic mutations in the DYSF gene encoding dysferlin, a protein necessary for muscle cell membrane repair. LGMD2B is a progressive muscular dystrophy that leads to loss of ambulation in adults and deterioration of daily activities.
The Berlin group used CRISPR/Cas9 directed to a recurrent frameshift mutation in exon 44 of the DYSF gene to generate indels that, when repaired by non-homologous end-joining (NHEJ), would introduce the correct nucleotide and restore functional dysferlin production in a proportion of cells. With that approach, they successfully edited induced pluripotent stem cells (iPSCs) and muscle stem cells from affected individuals and tested the strategy in a purpose-generated mouse model. These mice harbour the human DYSF exon 44 sequence with and without the target mutation (hEx44).
After editing ex vivo-isolated muscle stem cells from those mice, the researchers transplanted them into the tibialis anterior of homozygous hEx44mut mice. Remarkably, donor muscle stem cells contributed to the stem cell niche in recipient muscles, indicating that they could also contribute to sustained muscle regeneration and dysferlin production (3).
Cell transplantation has practical limitations; nevertheless, the researchers note that edited cells could be transplanted locally in one or two specific muscles, for example the finger flexors that are affected in LGMD2B and are important for daily activities, but the approach will not offer a complete cure. Encouragingly, this ex vivo gene editing strategy demonstrates promising safety with minimal off-target editing and low immune response to the reframed dysferlin.
Autologous gene-edited muscle stem cells, which is at the basis of MyoPax GenPHSats platform, proposes an interesting and complementary approach to in vivo gene editing using viral or non-viral vectors to pack the CRISPR/Cas9 components and draws attention back to regenerative medicine using stem cells, which has been a very active research topic since the early 2000s. Several clinical trials have evaluated different sources of muscle stem cells to treat muscular dystrophies, mainly DMD, both by local injection and by systemic delivery. However, difficulties in obtaining large enough numbers of pure myogenic cells, along with other challenges, rendered these approaches less attractive for therapy compared to the improved gene therapy options and gene editing.
The potential of mutation-agnostic approaches for muscular dystrophies
Gene silencing and interference approaches
Considering the immense genetic heterogeneity of muscular dystrophies even within the same causative gene, mutation-agnostic approaches are highly attractive because they can potentially benefit a larger proportion of patients and therefore, they will also more cost-effective. The possibilities are almost endless, provided the molecular defect at the genomic and transcriptomic level is well understood and a compensatory or inhibitory mechanism has been identified.
The following examples illustrate the potential of CRISPR-based programmable gene modulation for muscular dystrophies as recently reviewed by Capelletti et al. (4).
In dominant facioscapulohumeral muscular dystrophy (FSHD1), a contraction of D4Z4 macro-satellite repeats associated with the DUX4 gene is responsible for loss of repressive epigenetic marks. Consequently, DUX4, which is normally transcriptionally repressed in somatic tissues, is aberrantly expressed, causing dysregulation of muscle fibre signalling, apoptosis, atrophy and muscle tissue dysfunction.
Various CRISPR interference (CRISPRi) systems have been used to reduce the expression of DUX4, e.g., where a catalytically-dead form of Cas9 (dCas9) bound to known transcription repressors (e.g., KRAB, HP1α, or HP1γ, the repressor domains of SUV39H1 SET and of MeCP2 TRD) are directed to the DUX4 promoter, exonic regions or DUX4 regulators to silence DUX4 expression. The downregulation of DUX4 was reported in FSHD patient-derived myocytes and in a disease mouse model in which the CRISPRi components were administered using an AAV vector (5).
At the forefront of epigenetic efforts to treat FSHD is Epicrispr Biotechnologies, which has announced plans to start a Phase I trial of its lead programme EPI-321 in New Zealand in 2025 after obtaining FDA clearance for its IND application in April 2025. EPI-321 consists of a compact dead Cas9 (dCas9ONYX) protein fused to gene-suppressor modulators that re-methylate the DUX4 locus, along with a gRNA targeting D4Z4, all packaged in an AAVrh74 vector.
Another candidate for treatment via CRISPRi is Myotonic dystrophy (DM1), the most common muscular dystrophy in adults. DM1 is characterised by progressive muscle weakness, myotonia (sustained muscle contraction), cardiac conduction abnormalities and metabolic alterations. DM1 results from an expansion of CTG repeats in the 3’ untranslated region of the serine-threonine DM1 protein kinase gene (DMPK). This expansion leads to the accumulation of abnormal DMPK transcripts that sequester Muscleblind-like (MBNL1) splicing factors, causing the formation of characteristic foci within the nucleus and globally altering transcription.
Researchers at the University of Florida reported in 2017 that directing the dCas9-KRAB interference system to the DMPK locus achieved approximately 80% silencing, recovery of the normal transcriptome and spliceosome and functional contraction in DM1 muscle cells. In that same study and using AAV6 to transduce dCas9 and gRNAs in HSA mice, a model of DM1, researchers ameliorated several of the molecular and clinical hallmarks of the disease (6).
A more direct strategy than gene silencing, which has also been evaluated for DM1, is the excision of the CTG expansion using a pair of gRNAs. This approach was explored in DM1 cells and mice by Izzo and colleagues. For in vivo injection in DMSXL mice, which is an established model for DM1, those researchers placed two carefully selected sgRNA sequences flanking the CTG repeats in a self-complementary AAV vector (scAAV). Unlike single-stranded AAV, scAAV will self-associate after infection to form one double-stranded DNA (dsDNA) unit that is ready for immediatereplication and faster gRNA transcription. Because of the smaller loading capacity of scAAVs, the SpCas9 sequences were placed in a separate, muscle-tropic AAV vector (AAV Myo) under the control of the CK8 promoter (7). This approach resulted in effective gene editing and improved molecular and phenotypic alterations in mice, with fewer unwanted effects than systemic therapy, by circumscribing the editing to skeletal muscle and differentiated muscle cells.
Gene activation and compensatory approaches
Instead of repressors, dCas9 can be bound to gene expression activators and in this way induce the expression of a gene or genes to functionally compensate for the defective gene(s). This is the rationale behind work carried out at the University of Pittsburgh, where researchers used a dCas9-VP64 activator fusion and gRNAs targeting the LAMA1 gene promoter to upregulate its expression and compensate for the deficiency of laminin-alpha 2 chain which is encoded by the closely related lama2 gene. Laminin-alpha 2 is a component of laminin-2 or merosin, a key basal lamina protein of muscle fibres, peripheral nerves and other anatomical structures. Recessive mutations in the human LAMA2 gene lead to a severe form of Congenital muscular dystrophy (MDC1A). Remarkably, these studies showed that prevention of muscle fibrosis and reversal of disease progression could be achieved when the CRISPR activation components were administered both in pre-symptomatic and symptomatic lama2-deficient mice (8). Additionally, this approach was tested in human fibroblasts derived from MDC1A patients, confirming the potential of LAMA1 upregulation as a viable therapeutic strategy (9).
LAMA1 upregulation in skeletal muscle is the rationale behind MDL-101, an epigenetic drug candidate being developed by Modalis Therapeutics. Using proprietary CRISPR-GNDM® technology and skeletal-muscle tropic AAV9 vectors, Modalis reported durability, safety and efficacy data in mouse models and non-human primates, despite a positive immune response against Cas9 protein, paving the way for future clinical trials in humans. MDL-101 received Rare Pediatric Disease and Orphan Disease designations from the FDA for the treatment of MDC1A in 2024. Modalis Therapeutics’ pipeline also includes pre-clinical programmes for DMD, facioscapulohumeral muscular dystrophy and DM1.
Delivery challenges and emerging solutions
Safe delivery of gene-editing reagents (and in general of nucleic acid therapeutics) to skeletal muscle, in sufficient amounts to achieve clinical endpoints, is a major bottleneck for gene-editing based treatments. For example, the limited packing capacity of AAV vectors, which are the most widely explored delivery system to date, hinders their use. That may change with the emergence of more compact Cas systems, such as the recently described NanoCas by Mammoth Biosciences which successfully edits the dystrophin gene in mice and human primates (10).
Beyond AAV vectors, a number of very attractive, non-viral delivery vehicles have emerged in recent years; these include lipid, synthetic and inorganic nanoparticles and extracellular vesicles, which can deliver Cas proteins or Cas mRNA together with guide RNAs (recently reviewed in 11). However, examples of preferential targeting of skeletal muscle using these non-viral carriers after systemic treatment (e.g., intravenous injection) are still limited.
Timing is another key issue for the success of gene editing for muscular dystrophies. Most muscular dystrophies, like many rare diseases, manifest at birth or in childhood, and it is expected that the earlier the therapeutic intervention, the larger the impact on patients' health and quality of life.
In this line, researchers at the University of California argued that efficient delivery of mRNA, and thus, potentially gene editing, could be achieved using lipid nanoparticles at the in utero stage when the permeability of the blood vessels is higher, target tissues are lighter, and the immune system is more tolerogenic. Following this rationale, they intrahepatically injected mouse embryos with mRNA encoding the enzyme Cre recombinase. They used transgenic mice (Ai9) whose tissues emit red fluorescence when the Cre mRNA is translated within transfected cells. They found that 4 days after mice were born, skeletal muscle, diaphragm and the heart contained a significant proportion of positive-transfected cells (between 24 and 50% approximately). However, the editing efficiency was very low (less than 1% in kidney) when Cre mRNA was replaced by Cas9 mRNA and gRNAs (12). While still in its early stages, prenatal editing is a developing field that may hold future potential.
Conclusion
While gene addition therapy shows encouraging progress in clinical development for certain recessive muscular dystrophy forms—including SRP-9003 for LGMD2E/R4 (developed by Sarepta Therapeutics) which completed enrolment late in 2024—gene editing brings hope to individuals and families affected by the spectrum of muscular dystrophies.
Precise gene editing or gene expression modulation using CRISPR/Cas systems could address a broad range of mutations while raising fewer concerns about genotoxicity. On the other hand, developing safe delivery platforms remains perhaps the biggest challenge yet for systemic editing of skeletal muscles.
References:
- Chamberlain JS, Robb M, Braun S, et al. Microdystrophin Expression as a Surrogate Endpoint for Duchenne Muscular Dystrophy Clinical Trials. Hum Gene Ther. 2023 May;34(9-10):404-415.
- Lek A, Wong B, Keeler A, et al.Death after High-Dose rAAV9 Gene Therapy in a Patient with Duchenne's Muscular Dystrophy. N Engl J Med. 2023 Sep 28;389(13):1203-1210.
- Escobar H, Di Francescantonio S, Smirnova J, et al. Gene-editing in patient and humanized-mice primary muscle stem cells rescues dysferlin expression in dysferlin-deficient muscular dystrophy. Nat Commun. 2025;16:120.
- Capelletti S, García Soto SC, Gonçalves MAFV. On RNA-programmable gene modulation as a versatile set of principles targeting muscular dystrophies. Mol Ther. 2024;32(11).
- Himeda CL, Jones TI, Jones PL. Targeted epigenetic repression by CRISPR/dSaCas9 suppresses pathogenic DUX4-fl expression in FSHD. Mol Ther Methods Clin Dev. 2020 Dec 10;20:298-311.
- Pinto BS, Saxena T, Oliveira R, et al. Impeding Transcription of Expanded Microsatellite Repeats by Deactivated Cas9. Mol Cell. 2017 Nov 2;68(3):479-490.e5.
- Izzo M, Battistini J, Golini E, et al. Muscle-specific gene editing improves molecular and phenotypic defects in a mouse model of myotonic dystrophy type 1. Clin Transl Med. 2025 Feb;15(2):e70227.
- Kemaladewi DU, Bassi PS, Erwood S, et al. A mutation-independent approach for muscular dystrophy via upregulation of a modifier gene. Nature. 2019 Jul;572:125-130.
- Arockiaraj AI, Johnson MA, et al. CRISPRa-induced upregulation of human LAMA1 compensates for LAMA2-deficiency in Merosin-deficient congenital muscular dystrophy. bioRxiv [Preprint]. 2023 Mar 7:2023.03.06.531347.
- Rauch BJ, DeLoughery A, Sper R, et al. Single-AAV CRISPR editing of skeletal muscle in non-human primates with NanoCas, an ultracompact nuclease. bioRxiv. 2025.01.29.635576.
- Cavazza A, Molina-Estévez FJ, Reyes ÁP, et al. Advanced delivery systems for gene editing: A comprehensive review from the GenE-HumDi COST Action Working Group. Mol Ther Nucleic Acids. 2025 Jan 17;36(1):102457.
- Gao K, Li J, Song H, et al. In utero delivery of mRNA to the heart, diaphragm and muscle with lipid nanoparticles. Bioact Mater. 2023 Feb 17;25:387-398.
About the author
Cecilia Jimenez-Mallebrera, based at Barcelona's Sant Joan de Déu Pediatric Hospital, specialises in translational research in neuromuscular diseases. Her work integrates advanced therapies and biomarker discovery for paediatric neuromuscular conditions. Following a Biology degree from the University of Navarra and a PhD in Genetics from University College London, studying the utrophin gene, she completed postdoctoral work at the Dubowitz Neuromuscular Centre in London, focusing on congenital muscular dystrophies. Since establishing her research group in 2010, she has pioneered CRISPR/Cas9 applications for muscular dystrophies and implemented serum GDF-15 as a biomarker for mitochondrial diseases. Her research group participates in various clinical trials for Duchenne Muscular Dystrophy, Spinal Muscular Atrophy, Pompe´s Disease and other muscle diseases and contributes to several research networks such as the EU ERDERA consortium and the COST Action on Gene Editing, GenE-HumDi, as well as the Spanish Biomedical Research Network on Rare Diseases, CIBERER. Her research is supported through public institutions (Instituto de Salud Carlos III, Spain), private organisations (Fundación Noelia), and various research consortia, including the EU ERDERA and CIBERER networks. Read more about her research here.
Tags
AnalysisArticleNewsStickyCongenital Muscular Dystrophy, CMDDuchenne Muscular Dystrophy, DMD
CLINICAL TRIALS
Sponsors:
Suzhou Maximum Bio-tech Co., Ltd.