Prime Editing: CRISPR’s Final Evolution
Welcome back to Making the Cut! In the last weeks, we went on a bit of a detour with a 3-episode special on CAR-T cells and how CRISPR is used to not only produce CAR-Ts but also superpower them in their fight against cancer. While that was a lot of fun, we’re sure that some of you are looking forward to going back to the nitty and gritty of CRISPR, and we’re happy to oblige!
So on today’s episode, we will talk about one of the most fascinating CRISPR iterations, Prime Editing. And we know you may be thinking about Base Editing, which we talked about some weeks ago, and wondering how it relates to this. Well, think of it like a Pokémon evolution: CRISPR is like Charmander, all cute and with lots of potential, but without full control of his powers. Now Base Editing would be Charmeleon, the first evolution, more powerful than CRISPR but still limited (and yes, we know Charmeleon is kind of a temperamental teenager, it’s just a metaphor). Today we get to meet the Charizard of CRISPR technologies: not only is it way more powerful than its previous versions, it’s also way cooler!
Prime editing: DNA integration without double-strand breaks
Before we get to how prime editing works, we need to refresh a couple of concepts:
First of all, in episode 11 we explained in detail how CRISPR can be used to introduce external DNA sequences, which we called targeted integration. You may remember that we mentioned that generating double-strand breaks (DSBs) that open the DNA was necessary for this process. And we have already talked about the risks of those DSBs. So the logical question would be “Is it possible to perform targeted integration without a DSB?” And the answer is yes, but we’ll get to it in a minute.
The other thing you need to remember came in episode 9, where we explained what Charmeleon - sorry, Base Editing- is. If you haven’t read that article, we recommend you do so, because a lot of the concepts will be similar. Short recap: base editing consists of fusing an inactive Cas9 (dCas9 or dead Cas9) to an enzyme called a deaminase. Cas9 guides the deaminase to a target region where it can chemically transform one base into another. Got it? Good!
Now what is prime editing and why is it an evolved version of Base Editing? Well, the main limitation of base editing is that we can’t do anything else than transform one base into another. While this already has tons of applications, as we saw in episode 10, being able to change only one base at a time presents significant limitations. What if we need to substitute multiple bases, or even delete or introduce a DNA sequence? Initially, only DSB-based methods allowed us to do that. However, this all changed in 2019, when the lab of David R. Liu (who else?) introduced Prime Editing.
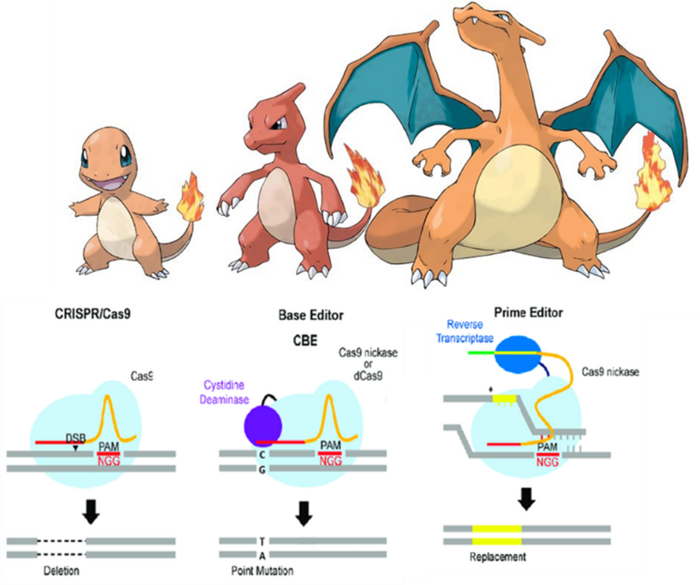
Evolving the concept: from base editing to prime editing
The objective was clear: how do we introduce or delete DNA sequences without actually cutting the DNA? For most people, that question seemed like a riddle without an answer. But the lab of David Liu had an idea. They based this idea on their previous design, base editing, which as we said fuses dCas9 to a deaminase enzyme. They decided to evolve this design by replacing this deaminase with a different type of enzyme: a reverse transcriptase.
Now reverse transcriptases don’t really occur naturally in our cells, but they are instead derived from RNA viruses. These pesky parasites are very interesting because they do not have a genome made of DNA, but instead of RNA. And those two, while similar, are not exactly compatible. So, in order to reproduce, RNA viruses need to enter a cell and then transform their RNA genome into DNA so that it can be inserted into the cell’s DNA. The enzyme responsible for this is a reverse transcriptase, called reverse because the transcriptases in our cells make RNA from DNA and this one… well, does the opposite thing.
And what does a virus enzyme have to do with this? Well, having a reverse transcriptase attached to dCas9 means that, when our protein complex (which we will call a prime editor) reaches the target sequence, we can make a DNA molecule right in situ. Kind of that hipster friend who always has their Polaroid camera with them, but useful.
And we know you’re asking “but if the reverse transcriptase transforms RNA to DNA, where does the RNA come from?” And that is a great question, as usual! You see, the genius of the prime editor design is that dCas9 already has an RNA molecule with it. Remember our friend the sgRNA? We know you do. Well, the scientists at the Liu lab decided to bind this sgRNA to a second RNA molecule that acted as the template for the modification they wanted to introduce. The new molecule formed by putting together sgRNA and editing template is called a prime editing gRNA (or pegRNA, yay creativity!) This intelligent design guarantees that, wherever the prime editor protein complex goes, the editing template is always going to be right there. Just like your friend’s man purse, the one where he keeps the instant film.
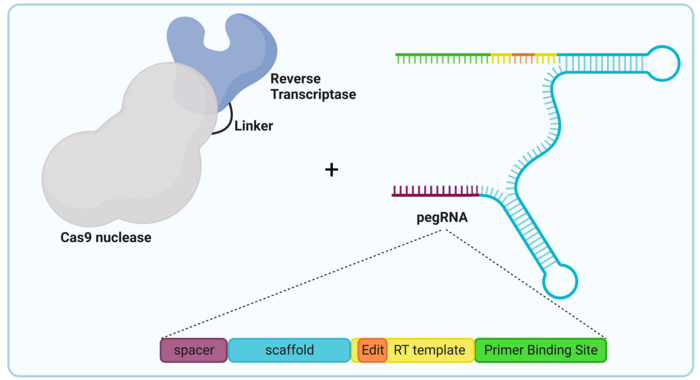
Tricking the cell with intelligent design
Okay, we got the components: dCas9, reverse transcriptase and pegRNA. So how does this actually work? This is a complex concept involving highly specific DNA dynamics that still sounds like science fiction to us after five years, but we will try to summarize the gist of it. First of all, when the prime editor binds to its target sequence, the two strands of the DNA will be separated.
The pegRNA’s editing template includes a primer binding site (PBS), which is a region with homology to our target sequence and will bind to one of the DNA strands, keeping everything in place. After the PBS, there is the template that will be transcribed from RNA to DNA by the reverse transcriptase. This template includes homology arms to the target sequence, as well as the edit we want to introduce: either the same sequence lacking some bases -for a deletion – or a new sequence -for an insertion-.
When the new DNA molecule is produced by the transcriptase, it will stay attached to the PBS, and thus to the open DNA strand. Then there will be some shenanigans that will confuse the cell’s DNA repair mechanisms, including cutting the other DNA strand, so that it believes that whatever DNA we have added through the prime editor is the actual cell’s DNA. This will make sure that this new DNA is kept when the DNA is repaired, leading to our desired edit.
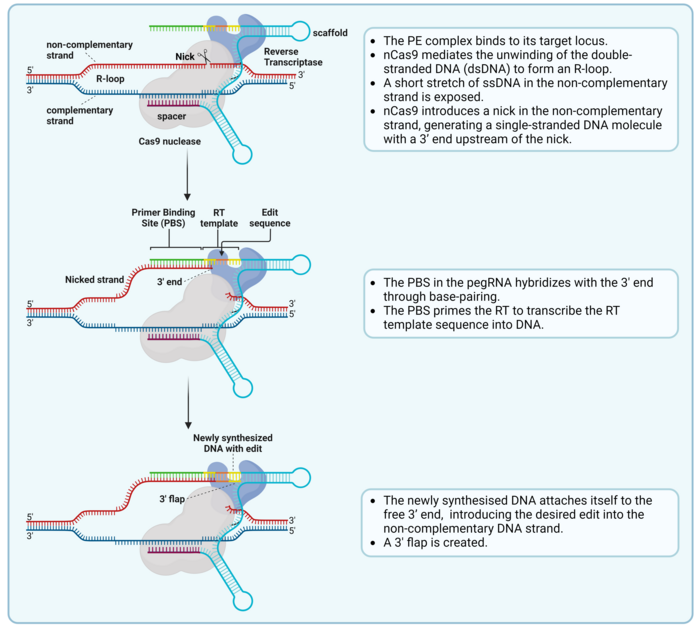
While the whole process of how prime editing works is a bit too complex to explain here, CRISPR Medicine News has a great explainer article, written by our Manel Llado Santaeularia, where you can find out more about the mechanism behind this fascinating technology. For now, we hope that you understood the main concept of how Prime Editing works!
Next episode, we will look at how this technology can be used to cure diseases, and also at how scientists are already working on a new evolution for it (MegaCharizard, anyone?) Until next time!
To get more CRISPR Medicine News delivered to your inbox, sign up to the free weekly CMN Newsletter here.