Acoustofluidics - Sounding out New Ways to Delivery Gene Therapies
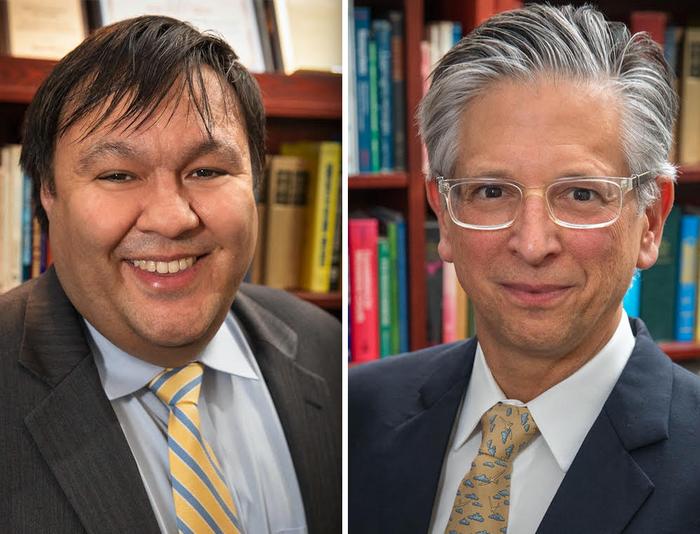
I recently interviewed Steven Jonas and Paul Weiss to hear about how a paediatrician and a nanoscientist joined forces to tackle what may be the greatest roadblock for the clinical translation of cellular and gene therapies.
Steven Jonas takes the lead: »My clinical expertise is within bone marrow transplantation and the applications of different types of cellular therapies. When I am attending on our inpatient service I take care of paediatric patients who are receiving such therapies on a daily basis. One of the discussions that often comes up with my physician-scientist mentors and colleagues is the issue of delivery.»
He explains the delivery challenge: »Whether we're talking about a gene editing therapy, a stem cell transplant, or a cancer immunotherapy, there's a thread that connects them together. And that is the need to deliver some kind of gene or gene editing package to many cells efficiently, quickly and cost effectively. A lot of these therapies right now are extraordinarily expensive, and take a long time to make.»
With a PhD in materials science and real-life appreciation for the gene delivery problem, Steven challenged Paul Weiss’s lab about 4 years ago to develop a high-throughput method to deliver genetic payloads to cells efficiently, safety, and cheaply and on a large scale. The initial results of this work were recently published in the journal PNAS.
»We essentially wanted to set up assembly lines for therapeutic cells», said Steven Jonas.
The ‘Billion Per Hour’ Criterion
Paul Weiss is a nanoscientist whose lab includes experts in chemistry, physics, biology, medicine, electrical engineering, mechanical engineering, bioengineering, and more. Prior to moving to UCLA 10 years ago, his lab had worked with artificial cell membranes and devised ways to create transient pores, but without ever finding a translatable application for their findings at the time.
When Steven approached the Weiss lab, they had a good reason to work with membranes again. »We were challenged with a ‘billion per hour’ criterion. That is approximately the number of cells needed for one stem cell treatment for a 12 kilogram child», explains Paul Weiss.
Acoustofluidic Delivery of Plasmids to Human Cells
Inspired by some of their previous work, Jonas and the Weiss group explored a handful of different approaches and eventually set about developing an acoustofluidic device that could deliver DNA to human cells with high throughput.
In their setup, which is illustrated in Figure 1, Jurkat cells (which are typically used as a model for immune cells) and a DNA substrate (plasmid or loose DNA) are simultaneously passed through a square glass microcapillary in the presence of a saline buffer solution. The capillary sits on a piezoelectric transducer that emits acoustic pressure waves that are felt by the cells inside the capillary. These waves cause physical shearing of the cell membranes against the capillary wall, creating membrane pores that allow the DNA cargo to enter. Cells are then collected at the outlet end of the capillary.
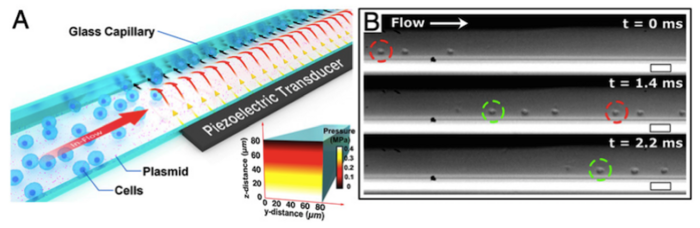
The researchers used microscopy and high-speed camera recording to track the cells within the capillary during the acoustofluidic treatment. This revealed that the applied acoustic forces pushed the cells against the capillary walls, leading to sonoporation, which ultimately permeablised the plasma cell membrane, allowing DNA to enter.
Intracellular delivery of fluorescently labelled DNA (Cy3-DNA) or a plasmid expressing green fluorescent protein (GFP) was tracked via confocal microscopy, which revealed the presence of DNA around the nucleus, in the cytosol and on the cell membrane. By plotting the relative fluorescence intensity of the DNA in the various locations the researchers could deduce that the DNA was trafficked by endosomes as aggregates from the cell membrane into the cell.
Comparison to Other Strategies
Viral-based delivery is standard for existing gene therapies, but these methods are very costly and somewhat prone to off-target events that reduce their efficacy and raise concerns about their safety. Newer therapies based on CRISPR technology are expected to overcome some of these drawbacks – they are programmable and lead to permanent i.e. stable genetic changes – but they still rely primarily on viral vectors to delivery Cas endonucleases and other necessary CRISPR components to cells, which often exceeds the size limitations of viral vectors.
Non-viral delivery approaches also exist, e.g., nanoparticle delivery, microinjection, electroporation and lipofection, but these also vary in efficacy and throughput. In their current state of progress none of these strategies can meet the billion per hour criterion.
A technology called cell squeezing bears the closest resemblance to the new acoustofluidic setup described here. This makes use of constricted microchannels to form pores in cell membranes through which molecules e.g., plasmids can enter, and it has so far shown promise for the delivery of CRISPR components to human cells. However, despite some similarities to cell squeezing, acoustofluidic delivery is unique, as Paul Weiss explains:
»The acoustofluidic approach turned out to be the most promising of all the approaches we tried. We didn’t have to use any channel coatings, so there is no need to clean the cells after the treatment, and the channel walls don’t clog because we don't put the cells through any physical constriction. We basically define that constriction acoustically with control into the bounds of the sounds of walls.»
Proof of Concept – Gene Delivery to Primary Human Cells
To fine-tune the setup, the group optimised the acoustic frequency and amplitude to obtain maximal cell viability, which was remarkably above > 90 % immediately after delivery, and remained above 75 % 72 hours later. Tony Huang PhD, Professor of Mechanical Engineering and Mechanical Science at Duke University, and expert in acoustofluidics was also involved in the project, which ultimately resulted in an unprecedented throughput of 200,000 Jurkat cells per minute in a single channel. This is relatively straightforward to scale up by using many parallel channels.
To test the device’s potential in therapeutically relevant cell types, the group attempted to deliver a GFP-expressing plasmid to primary peripheral blood mononuclear cells and haematopoietic stem cells using the same parameters that gave optimal results in Jurkat cells. They observed increasing GFP expression in both primary cell types in the 72 hours post delivery, with viability above 85 %. Overall, the delivery efficiency was lower for primary cells but optimisation of device parameters for each cell type is expected to enhance delivery efficiency and transgene expression.
What’s Next?
This work led to a brand new platform for gene delivery, and this throws up a lot of questions about its potential for the development of therapeutics.
Future experiments will reveal how broadly transferable the new technology will be to disease-relevant cell types. It will also be exciting to see whether there will be size limitations to the DNA that can be delivered acoustofluidicly, and what other cargoes might be able to enter the cell this way. The group plans to examine the delivery of CAR-T constructs as well as other cargo types for gene therapies, e.g., therapeutic proteins. Steven Jonas’s focus for the immediate future will be paediatric cancer immunotherapy and bone marrow transplant therapies.
Now that the team has met the billion per hour criterion, when are the children likely to benefit?
“We want to get there as quickly as possible. By expanding our collaborations with physician scientists in the cellular therapy space, optimising the platform to our clinical targets and cell types, and improving our understanding of how to best package and to deliver the therapeutic packages, we want to provide a toolkit for our colleagues that enables the accelerated discovery and implementation of new therapies.”
Link to original article in PNAS:
Acoustofluidic sonoporation for gene delivery to human hematopoietic stem and progenitor cells.
Tags
CLINICAL TRIALS
Sponsors:
Poseida Therapeutics, Inc.
