Decoding Guide RNA Rules for Cas13
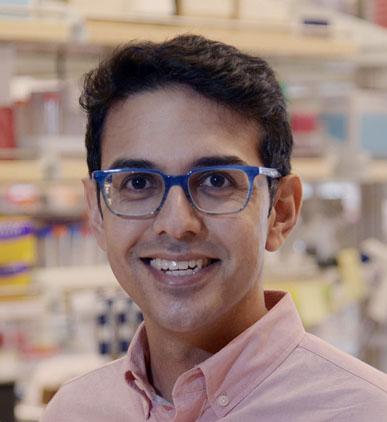
The RNA-targeting endonuclease Cas13 has recently attracted a lot of attention within molecular diagnostics. But Cas13 has another exciting aspect that has received little attention: the ability to selectively target cellular RNAs and influence gene expression without making permanent genetic changes.
Findings presented in a new study by Neville Sanjana and colleagues at the New York Genome Center and NYU mean that scientists can now target virtually any RNA in the human genome for high-throughput screen using CRISPR technology based on Cas13. The RNA-targeting enzyme is much more specific than the currently available RNA-targeting technologies such as RNAi.
»We've actually predicted Cas13 guides for all genes in the genome. And using this, we’ve developed a user-friendly, web-based interface that accepts any RNA sequence and returns an optimal guide RNA sequence. It can work for any organism, for any RNA,« says Neville Sanjana.
The researchers describe how they assessed the knockdown efficiency of almost 25,000 distinct guide RNAs (gRNAs) to infer the ‘rules’ for optimal gRNA design.
Using their new data, the researchers were able to train a machine-learning model to predict gRNAs for every protein-coding transcript in the human genome. I caught up with Neville Sanjana earlier this week to hear more about the work.
It Started With CRISPR-Cas9 Screens
As a postdoctoral fellow, Neville Sanjana worked in the lab of Feng Zhang at the Broad Institute. Here, he co-developed the very first genome-wide CRISPR Cas9 screens that exploited the programmable nature of the DNA-editing Cas9 endonuclease to target the entire human genome.
Despite the many advances that have emerged from the screens on protein-coding genes, it turned out that DNA editing and mutagenesis is less efficient for targeting many kinds of non-coding RNAs.
According to Neville Sanjana, non-coding RNAs are emerging as important regulators of many biological processes, including gene expression, translation and post-translational modification. Their roles in health and disease are highly complex, and further insights may hold the key to therapeutic targets for currently untreatable diseases. The difficulties in targeting these with CRISPR-Cas9 became the motivating factor in developing a new RNA-targeting high-throughput screen platform.
Cas13 Overview
Cas13 enzymes are Type VI CRISPR (clustered regularly interspaced short palindromic repeats) enzymes that have recently been identified as programmable RNA-guided, RNA-targeting proteins with nuclease activity that allows for target gene knockdown without altering the genome. This property makes Cas13 a potentially significant therapeutic for influencing gene expression without permanently altering genome sequence.
A Case for CRISPR-Based RNA-Targeting Screens
Today Sanjana is heading up his own lab, where a major ambition is to identify novel functional elements in the human non-coding genome. The idea with the latest work was to take advantage of the RNA-targeting ability of Cas13 to perform massively-parallel screens on non-coding RNAs. But, as Neville Sanjana points out, RNA-targeting by itself is not new:
»RNA-targeting technologies have actually existed for a couple of decades now, e.g., RNAi. But those have had their own problems. In fact, the Cas9 screens became so popular at the time because the technologies that they were replacing, many of which were based on RNAi, were plagued with off-target activity and false-positives. In many cases, only a few of the top hits from any RNAi screen could be validated in subsequent work.«
Cas13 Is Less Hackable Than Cas9!
The lab initially set out to repeat what had been done with the first Cas9 screens at the Zhang Lab, just with Cas13 instead. The idea was to collect a large dataset on a defined panel of RNA targets, and use this data to train a machine-learning model that could predict gRNAs for any RNA target.
After testing three different Cas13 variants, they settled on a Cas13 known as RfxCas13d, which was originally isolated in Patrick Hsu’s lab from the bacterium Ruminococcus flavefaciens, and exhibits robust and efficient RNA knockdown efficiency and minimal off-target activity. They selected gRNAs for certain non-coding RNA targets, and found that some gRNAs worked well and others didn’t work at all, but it wasn’t obvious why. Had it been a Cas9 screen, they could have availed themselves of many of the different freely available gRNA prediction tools, but nothing similar existed for Cas13. So they set out to create it. But first, the researchers realised that they had to go back a few steps and figure out what constituted a ‘good’ gRNA for Cas13.
gRNA Design Insights from GFP Reporter Screens
To gather a large dataset that could be used to infer principles for optimal Cas13 gRNAs, the group systematically assessed the knockdown efficiency of thousands of gRNAs in a human cell line that expressed green fluorescent protein (GFP) and Cas13 fused to a nuclear localisation sequence.
gRNAs with 0, 1, 2 or 3 single-nucleotide mismatches relative to GFP’s coding sequence were compared by monitoring GFP expression, and the gRNAs that led to efficient knockdown were identified through a reduction in fluorescence.
These experiments revealed important insights about the regions of the gRNA that govern recognition of a target RNA, including a critical "seed" region that cannot tolerate mismatches between the gRNA and the target RNA.
The group then looked beyond the ‘seed’ region and used structural biology and mutational analysis to examine the effects of surrounding nucleotide context on knockdown efficiency, as well as the impact of gRNA folding and secondary structures.
In addition to the target-specific sequence, the team also optimised the RNA handle that Cas13 uses to recognise its gRNA: »Inspired by everything we had learned about Cas9 throughout the years, we tried to take the gRNA sequence and make it better using some of the same kinds of hacks that have been done with Cas9. In the end, we could only create one synthetic gRNA that was better than the natural one and it really improves performance of sub-optimal gRNAs. But 90% of what we tried was actually worse than what nature had come up with. In some ways, the gRNA rules for Cas13 seem to be less flexible than with Cas9!«
The team built all of these observations into an in silico model that was able to make predictions that largely agreed with the experimental results they had obtained. After further refinements, including testing of predicted gRNAs against a number of essential human genes, the model could correctly predict gRNA knockdown across numerous human targets. The refined model was then used to predict gRNAs for all protein-coding transcripts in the human genome and these predictions have been made available through a user-friendly web-based application called Cas13design.
Cas13design - A New Resource for the Research Community
Cas13 design is available to researchers through an interactive website and open-source toolbox to predict gRNA efficiencies for custom RNA targets and provides pre-designed guide RNAs for all human protein-coding genes.
Future Perspectives
The results of this work allow researchers to do something that was not possible before: to make targeted knockdowns of any RNA in the human genome. This is a very exciting prospect that raises many questions particularly with respect to therapies, such as: how long will the RNA knockdown last?, what will be the optimal way to deliver Cas13 and gRNAs to cells?, and what diseases are likely to benefit from a Cas13 therapy?
»I like to think it's a platform technology like Cas9. I think there will be a broad set of diseases for which CRISPR therapies are relevant. For some it will be preferable to target RNA and for others a one and done DNA-based genome editing approach will be better,« says Neville Sanjana.
Before that happens several challenges have to met. For instance, the lab is now working on improving RNA and Cas13 stability by chemically modifying Cas13 gRNAs and Cas13, and they also have plans to test some of the emerging nanoparticle-based delivery methods for targeting the right cell or organ type.
And What About Non-Coding RNAs?
The project began with non-coding RNA's and Sanjana is hoping to use their new platform to carry out non-coding RNA screens as well.
»If we can find non-coding RNAs with disease relevance, we may be able to target them and modulate their expression with Cas13. That's very difficult with traditional CRISPR genome editing. In long non-coding RNAs, a small indel is not disruptive to the reading frame and doesn’t trigger nonsense-mediated decay in the transcript. In this situation, it may be better to knock down the entire RNA.«
Neville hopes that the new technology can reveal important insights into what he calls the dark matter of the genome: the non-coding RNAs that exist in much larger numbers than protein-coding RNAs.
»We have no idea how many of these (non-coding RNAs) there are and until now there's been very few tools to interrogate them in a high throughput, reasonable, uniform way. I want to understand why these exist? How do they work? If they indeed do, how do they regulate protein-coding genes? And are they involved in specific diseases?,« he says.
Link to original article in Nature Biotechnology:
Massively parallel Cas13 screens reveal principles for guide RNA design.
The Sanjana Lab
The Sanjana Lab, headed by Prof. Neville Sanjana, PhD, is located in The New York Genome Center and at the Department of Biology at New York University. The lab works at the interface of bioengineering, neuroscience and cancer and takes a multidisciplinary approach to address important questions about the human genome, such as how we can use knowledge about genetics to target diseases such as autism and cancer. On a practical level, the lab uses everything from molecular cloning to genome-wide screens to machine learning to build new technologies that can uncover novel functional elements in the human genome.
Tags
CLINICAL TRIALS
Sponsors:
Intellia Therapeutics
