New, DNA-Dependent Gene Editing Technology Could Shift the Paradigm of Precise Editing
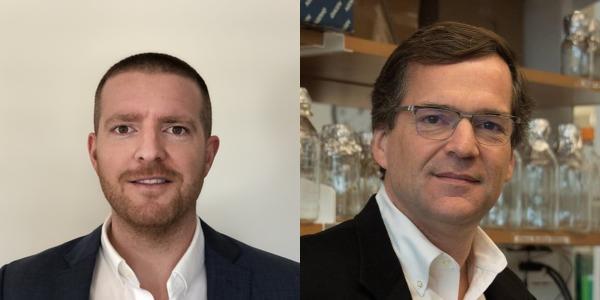
Prime editing is among the latest gene-editing modalities that can introduce precise edits in DNA without generating double-strand breaks. While it is a welcome addition to the gene-editing toolbox and is being pursued in therapeutic development programmes, prime editing presents some important limitations that scientists have been trying to address for the last five years.
For instance, the pegRNA molecules used in prime editing are difficult and expensive to chemically synthesise or laborious to clone, which hampers the crucial optimisation of prime-editing efficiency. Additionally, the reverse transcriptase (RT) enzymes used in prime editing are relatively error-prone and have low processivity, which may limit the precision and size of edits that can be introduced. Furthermore, RTs have a low affinity for dNTPs, which can impact prime-editing efficiency in non-dividing and differentiated cells.
To address these issues, two research groups led by Dr. Ben Kleinstiver at Mass General Hospital (MGH) & Harvard Medical School, and Dr. Erik Sontheimer at the RNA Therapeutics Institute (UMass Chan Medical School) have independently developed new approaches that build upon prime editing by replacing RT with another type of enzyme, namely a DNA-dependent DNA polymerase. This change permits the use of DNA instead of RNA as a template for editing, potentially addressing some of the main limitations of prime editing by allowing higher efficiency and adaptability.
We spoke with Dr. Kleinstiver and his PhD student Connor Tou and postdoctoral researcher Dr. Joana Ferreira da Silva, as well as with Dr. Sontheimer to learn more about the recent studies, and how their findings contribute to the greater gene-editing community.
The Kleinstiver group at MGH & Harvard Medical School has dubbed its new technology ‘click editing’, which was recently described in a preprint in BioRxiv.
»We have developed a new DNA writing enzyme architecture that harnesses the advantageous enzymatic properties of DNA-dependent DNA polymerases as well as the optimisation ease, manufacturing scalability, and chemical stability of separate DNA templates.«, says Dr. Ferreira da Silva, co-first author of that publication.
Dr. Sontheimer’s group at the RNA Therapeutics Institute published their findings in an article in Nature Biotechnology, in which they report a similar technology called DNA polymerase editing (DPE).
»By using a DNA polymerase, this system increases the fidelity of prime editing and could extend the length of the edits that we are able to make«, says Dr. Sontheimer.
Prime editing in brief
Prime editing combines the powerful DNA-scanning and sequence-identification capabilities of the CRISPR-Cas9 system with a reverse transcriptase enzyme, which uses an RNA template to synthesise a new single-strand DNA sequence and insert it into the DNA. Prime editing is considered to have a very desirable safety profile when compared to conventional CRISPR-Cas9-mediated gene editing approaches, because it allows for targeted DNA editing without the intentional generation of DNA double-strand breaks.
Read much more about prime editing in our recent article: 'Explainer: What Is Prime Editing and What Is It Used For?'
Learn more about the research from Ben Kleinstiver at the upcoming CRISPRMED24 Conference
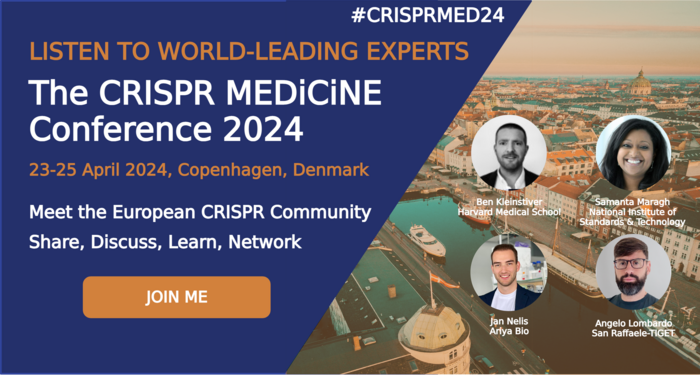
Using DNA polymerases and a DNA template for gene editing
As mentioned above, the two research groups set out to solve the same issues, and both rationalised that replacing the RT component of prime editors with a DNA-dependent DNA polymerase would offer significant advantages.
»There are some things about RTs that are not optimal for what we ask them to do. Our prime editors were not as good as we wanted in terms of fidelity, processivity and dNTP affinity. And we knew that there were a number of DNA-dependent DNA polymerases that are far superior in this regard,« explains Dr. Sontheimer.
Indeed, the increased dNTP affinity of DNA-dependent DNA polymerases (DDP) suggests higher activity in a broader range of cell types, including non-dividing and differentiated cells, where dNTP availability has recently been shown to be limiting for prime editing (1,2).
The second step was to separate the editing template from the gRNA, thus removing the need to synthesise a long pegRNA. However, this required that the template be tethered to the editing complex in another way.
The Sontheimer group decided to tackle this issue by fusing the DDP to an MS2 coat protein (MCP) that was able to recognise an MS2 stem-loop RNA domain inside the template and bind it. This meant that their template had to be made at least partially of RNA.
»The field of oligonucleotide therapeutics has been making DNA-RNA hybrids for many years. For instance, many antisense oligos that have entered clinical development, such as siRNAs for RNAi therapeutics, are similarly chimeric molecules, where you can have some RNA residues, some DNA residues, and even some 2’-methylribose or 2’-fluororibose residues. There's a whole universe of chemistry to work with when you build your molecule by solid phase synthesis,« explains Dr. Sontheimer.
The hybrid templates that they designed, hereafter referred to as DNA polymerase editing templates (DPETs), maintained the RNA MS2 stem-loop necessary to tether them to the polymerase through the MCP domain. However, the polymerase template region encoding the desired edit was made with DNA residues.
»We realised that we could make the template more stable by chemical synthesis, and that one of the things we could do was change the nature of the template region itself, to no longer rely on RNA as a template but use DNA instead,« says Dr. Sontheimer, who continues: »This is a great advantage compared to pegRNAs, because the efficiency of synthesis and purification can increase greatly when you incorporate fewer RNA residues.«
Finding the missing piece: How to bind a DNA molecule to an editing complex
Just 68km away, Dr. Kleinstiver's team was looking at the problem from another angle; they decided to get rid of any RNA in the template and use a fully DNA one. However, the issue was how to bind it to the editing complex. As it often happens in science, the solution came when least expected, as Joana Ferreira da Silva explains:
»The key moment was when Connor (Tou, co-first author) read a paper from Wendy Gordon’s lab at the University of Minnesota, which has pioneered a lot of the work with HUH endonucleases. When we read their work, we realised that those endonucleases might provide a way to covalently link a DNA template to the editor. This was the point when we got really excited about moving this technology forward.«
HUH endonucleases are able to covalently bind to specific single-stranded DNA sequences. By attaching the HUHe from porcine circovirus 2 (PCV2) to nCas9, Kleinstiver’s team was able to bind a single-stranded DNA template called click DNA (clkDNA) to their editor.
This innovation allowed the group to substitute the RT found in prime editors with a 3’-5’ exonuclease-deficient Klenow fragment from E. coli DNA polymerase I, generating the first generation of click editors (CE1), which achieved efficient gene editing in several loci and cell types. They also showed compatibility of their CE architecture with a wide range of DNA-dependent polymerases, including those with naturally high fidelity and processivity.
While both CPE and click editing approaches are very similar, it is worth pointing out that Dr. Sontheimer’s group delivered the two components of the editing complex, i.e., the nCas9 and the DDP, separately, while Kleinstiver and his team primarily fused the two proteins together (although they did also show that the DDP and nCas9 could be delivered separately).
Although both systems have been demonstrated to work similarly in vitro, there could be significant differences in terms of their in vivo delivery. For instance, the fused complex is too large to deliver easily, and so far only the AAV-based intein system has been shown to effectively deliver such complexes. Alternatively, the untethered editor complex can be delivered as two independent pieces, but since the DNA polymerase is recruited independently to the editing site, its concentration could be a limiting factor for efficiency. Another approach being explored by the teams is non-viral delivery, giving additional flexibility to encode the DPE or CE on mRNA, while providing a synthetic gRNA and DNA template in trans.
DNA templates are a more affordable and flexible solution
The editing process when using DPE and click editing greatly resembles that of prime editing. Indeed, the DNA templates have a composition that is very similar to that of the editing portion of pegRNAs. They, too, include a primer-binding site, a polymerisation template region with the intended edit and a 3’ homology arm. The main difference with prime editing is that both DPETs and clkDNAs are separate from the gRNA and include a sequence for binding to the respective editing complexes.
While template configuration is similar, the fact that these templates are made of DNA instead of RNA greatly facilitates their synthesis, as Dr. Kleinstiver explains:
»Synthesising long, modified pegRNAs is still really challenging, especially for lengthy insertions. In contrast, synthesising a long DNA molecule is relatively trivial, so it is simpler to order a long DNA oligo than a long RNA molecule. Additionally, there are different types of modifications you can add to DNA molecules to improve their stability, and thus click editing efficiency.«
In addition, using DNA templates allows the introduction of beneficial chemical modifications to the template. »We can make the residues in the PBS as 2’-methylribose, which increases their stability and resistance to degradation by nucleases,« explains Dr. Sontheimer.
According to Dr. Kleinstiver, using DNA as an editing template offers significant advantages when it comes to optimisation: »When we prime edit, we have to screen a lot of pegRNAs in order to optimise PE efficiency. For this, we have to undertake cloning efforts to construct molecules that include the gRNA and the template fused to each other. Cloning 100 or 200 pegRNAs is not trivial; it takes a lot of effort and time. With click editing, we don’t need a cloned template. For a particular target we generally use the same gRNA and CE1 editor, enabling us to perform large optimisation experiments using inexpensive and scalable clkDNAs, which we can order as concentration-normalised DNA oligonucleotides from commercial vendors.«
Considering the similarities between the two systems, Dr. Kleinstiver points out that: »Having another lab exploring a similar technological approach supports and validates the conclusions from our own work. The main difference between our platform and that of Dr. Sontheimer’s team is the type of template and how the polymerase gets recruited to the nCas9-bound site, but ultimately our methods are similar.«
DNA-based editing efficiency is comparable to prime editing
While both teams used different strategies, their results were quite similar. In both cases, the editing tools they developed managed to introduce the desired edits with a similar efficiency to that of the first prime-editing systems.
However, second-generation prime editors, which are much more widely used, include an engineered reverse transcriptase domain carrying several mutations that increase polymerisation efficiency. Although it is early days for click editing, Dr. Kleinstiver is very optimistic about what second-generation derivatives could yield:
»Second-generation prime editors are still significantly more efficient than CEs for now, which is exciting, because it means that we're likely to achieve higher levels of click editing if we can engineer the DDP to be more efficient.«
However, DPE and click editing already present some advantages when compared to prime editing. The most important one is the lack of scaffold integration, which is one recently discussed drawback of prime editing. Indeed, the attachment between the template RNA and the gRNA scaffold in pegRNAs can, in some cases, lead to unwanted integration of the gRNA scaffold in the edit site. The fact that DNA templates are not fused to the gRNA scaffold prevents this issue.
Additionally, the higher processivity of DDPs also bodes well for applying this technology to longer insertions, which are a significant limitation of prime editing.
»As we think about editing longer sequences, installing up to thousands of base pairs, the ability to do this with high fidelity and processivity becomes more and more important. The field is progressing to a point where we don’t only think about more precise genome editing for small mutations, but also about more generalisable approaches made possible by larger edits. Perhaps in the future, one or a few therapies could cover several patient sub-populations of a given genetic disease,« says Connor Tou.
As with any new technology, much optimisation will be needed to get the best out of this technology. As Dr. Sontheimer puts it: »There is still a long way for us to go to empirically optimise the outcome of the system. There’s the length of the primer-binding site, the length of the right homology arm, and various other knobs and dials that we can try to turn.«
Importantly, the use of easily synthesisable DNA templates allowed the Kleinstiver group to turn this optimisation, which is usually the most costly and time-consuming process, into a significantly easier and cheaper process, as Dr. Ferreira da Silva highlights:
»We managed to screen nearly a thousand different oligos in our paper, just by changing the composition of the oligo and the length of the template without any expensive chemical modifications. We think that click editing allows a very streamlined workflow to reach an optimal click DNA that then can be further modified to improve efficiency.«
Future steps for DNA-dependent gene editing
Both research groups agree on the next steps for this technology. As Dr. Kleinstiver puts it:
»We are focused on improving the efficiency of click editors. Once we improve their performance in cell culture, we're a lot more optimistic about getting them to work in vivo. Additionally, since these systems are pretty modular, we’re excited about the use of different Cas modules, either smaller orthologs or PAM variants that can read other parts of the genome.«
According to both groups, one main focus area for improvement is the possibility to introduce longer edits. Dr. Sontheimer explains it: »We've already seen increases in the efficiency of long insertions through various strategies that go beyond canonical prime editing, like Twin-PE or Jump-PE. We’re still not satisfied, but there’s been a gradual improvement in the lengths that can be worked on. I hope we are going to be able to apply some of the same ideas to our technology, and I'm optimistic that we will get there. But it's going to take a lot of work and a lot of clever advances.«
On the possibility of collaborating with each other, Dr. Kleinstiver says: »We’d be delighted to work together with Erik’s team rather than compete with him. I think that, together, we could build improved versions of either of these systems. There's a lot of really interesting things we can do.«
So far it seems like DDP-mediated gene editing will be the new kid in the ever-expanding neighbourhood of gene-editing technologies. Whether this new technology can reach the heights of its neighbours and become a staple in the gene editing field, only the future will tell.
Links to original articles discussed herein:
da Silva JF, Tou CJ, King EM, Eller ML, Ma L, Rufino-Ramos D, Kleinstiver BP. Click editing enables programmable genome writing using DNA polymerases and HUH endonucleases. bioRxiv [Preprint]. 2023
Liu B, Dong X, Zheng C, Keener D, Chen Z, Cheng H, Watts JK, Xue W, Sontheimer EJ. Targeted genome editing with a DNA-dependent DNA polymerase and exogenous DNA-containing templates. Nat Biotechnol. 2023
Other cited literature:
- Ponnienselvan K, Liu P, Nyalile T et al. Addressing the dNTP bottleneck restricting prime editing activity. bioRxiv [Preprint]. 2023 Oct 29:2023.10.21.563443. doi: 10.1101/2023.10.21.563443.
- Levesque, S., Verma, A., & Bauer, D. E. Nucleotide metabolism constrains prime editing in hematopoietic stem and progenitor cells. bioRxiv [Preprint]. 2023 Oct 22: 2023-10. doi.org/10.1101/2023.10.22.563434
Manel Llado is a gene editing expert and science writer/communicator based in Vienna, Austria
To get more CRISPR Medicine News delivered to your inbox, sign up to the free weekly CMN Newsletter here.
Tags
CLINICAL TRIALS
Sponsors:
Suzhou Maximum Bio-tech Co., Ltd.
Sponsors:
Zhejiang University