There Are Now Two Pushes to Treat Sickle Cell Disease With CRISPR
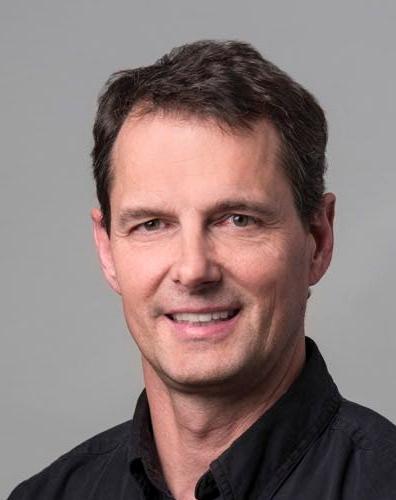
On December 14, the FDA approved Graphite Bio's Investigational New Drug (IND) application for a CRISPR Cas9 treatment for sickle cell disease (SCD). With that IND in hand, the biotech startup is free to pursue Phase 1 and 2 clinical trials, dubbed CEDAR, to test GPH101: The first therapy to directly correct the dangerous mutation that makes blood cells harden into a C shape.
SCD is a blood disorder wherein red blood cells are incapable of shuttling enough oxygen through the body because the haemoglobin protein that bonds to oxygen is deformed and inflexible. Globally, the mutations for SCD are rare, but they're more common among people with sub-Saharan African, Southern European, or Latin American ancestry. Sickle cells tend to die within 10 to 20 days rather than the typical 90 to 120-day lifespan of healthy cellsand are more likely to cause dangerous clots — both problems that can cause intense pain and organ damage over time.
»Sickle cell disease is a disease that's relentlessly destructive,« says Dr. Matthew Porteus, MDPhD, a professor of pediatrics at Stanford University and co-founder of Graphite Bio.
But if the upcoming clinical trials — which now await institutional review — go well, his company's therapy may prevent what can be a lifetime of painful episodes and medical complications, all by going in and correcting the mutation responsible. Specifically, that's a mutation in the beta-globin gene that codes part of the haemoglobin protein.
Gene-editing offers great promise
For Porteus, this IND is the culmination of decades of research into the genetic roots of SCD. Porteus was first inspired to go down this road during his medical training, when he was taken aback by the disconnect between how much scientists knew about Sickle Cell Disease and how little they could do to treat it. At the time, he could do little more than offer his patients opiates to treat the pain.
»It's been close to a 25-year journey,« Porteus says.
But the discovery of the CRISPR nuclease, Porteus adds, vastly accelerated the rate at which that journey progressed.
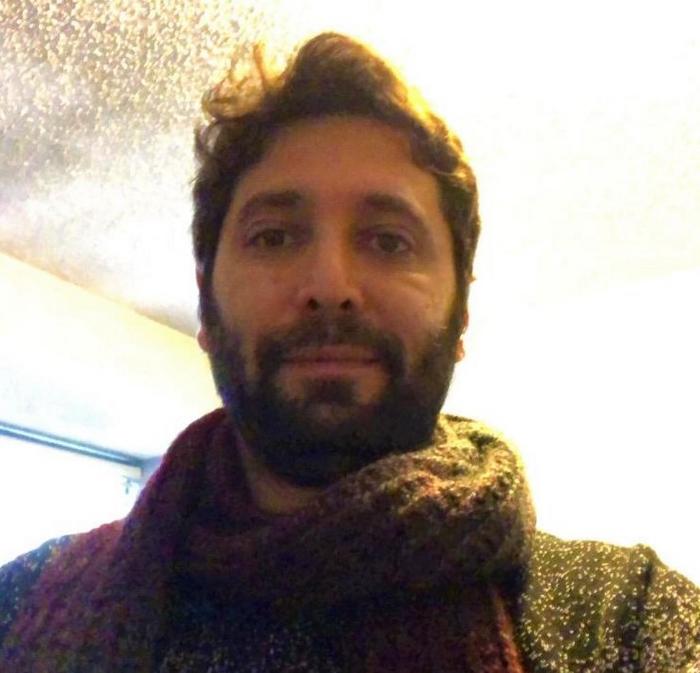
»The most promising aspect of the CRISPR technology is that it is theoretically available to all patients,« says Dr. Selami Demirci, Ph.D, a researcher at the National Institutes of Health who published a paper on potential CRISPR applications for SCD last year.
»When the processes are successfully translated into clinics, patients would not require a matched sibling donor as the patients’ own cells will be used for editing and correction approaches that eliminate rejection and graft-versus-host-disease problems.«
»Thus, genetic interventions offer great promise for patients who do not have a suitable donor,« Demirci adds.
Two different gene-editing approaches
Developing the gene therapy GPH101 puts Porteus and Graphite Bio in a pleasantly-crowded space, as far as applications for CRISPR go. Toward the beginning of December, Vertex Pharmaceuticals and CRISPR Therapeuticsshared clinical trial data from studies on CTX001, their jointly-developed CRISPR therapy for SCD.
To summarize, the data looks good — and experts celebrate it as a sign that a treatment for the disease could finally be on its way.
But most interesting is that the two groups take significantly different approaches to the same challenge. The Vertex/CRISPR group is using CRISPR to reawaken a fetal haemoglobin gene that typically goes dormant, whereas Graphite Bio is attacking the problem head-on, removing the mutation from the beta-globin gene and replacing it with a strand of healthy DNA.
Thankfully, when it comes to tackling such an important problem, there's no need for the scientists to get competitive.
»First of all, from a patient population standpoint, I think it's great that the patients might have choices,« says Porteus.
»There is the possibility that one approach, for a certain set of patients, might be better than another approach. We don't know that yet.«
As far as medical procedures go, the two treatments are surprisingly similar — the real differences come into play deep into each approach's methodology.
Editing stem cells from the patients
Both treatments are ex vivo cell therapies. That means that the actual gene editing is performed on cells that were removed from the patient's body in advance. Specifically, doctors pull the haematopoietic stem cells — responsible for forming red blood cells — from the patients' bone marrow, Porteus explains.
After letting them expand and proliferate for a few days, both teams inject the stem cells with their respective gene editing machinery through a process called electroporation, which uses an electric shock to form holes in the cell membrane.
»Our machinery has two parts to it. It has the CRISPR nuclease, which we deliver as a Cas9 protein, and the guide RNA. The guide RNA was made synthetically — that's what we call an RNP,« says Porteus.
That RNP locates and forms a break in a specific point of the beta-globin gene responsible for the sickle haemoglobin. Both CRISPR/Vertex team and the Graphite Bio team use an RNP, but they target different things.
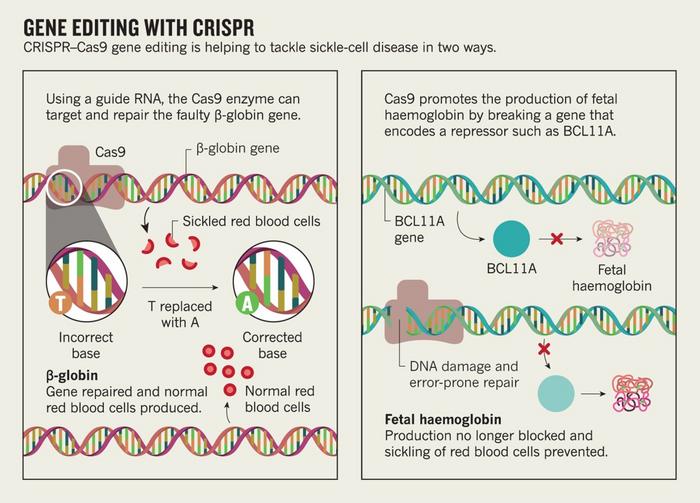
High efficiency and low genotoxicity
»Our second part, though, is we have to provide the cell a piece of DNA to repair the break,« says Porteus.
»In the CRISPR Therapeutics gene therapy, they're not targeting the mutation. They're targeting something else. They just make a break and let the cell repair itself.«
»So we provide to the cell an extra piece of DNA, a template DNA, and we do that actually using a nonintegrating virus. A virus that would normally just deliver its DNA to the nucleus but not integrate with it. The virus is called an AAV [adeno-associated virus]. So, the cell will use the donor DNA as a template, and take a copy and paste it into the break,« Porteus adds.
»I describe it as a copy and paste mechanism.«
That donor DNA contains the genetic sequence for a healthy version of that part of the beta-globin gene. That way, the stem cell that integrates and occupies that donor DNA will no longer have the sickle mutation that makes it generate deformed blood cells.
The process, Porteus explains, results in up to a 70 per cent correction of the sickle globin allele on haematopoietic stem/progenitor cells (HSPCs) with very low genotoxicity — meaning the treatment works fairly well on the part of the gene it's supposed to change and doesn't seem to affect other regions that ought to be left alone.
While scientists aren't sure what percentage of cells it's necessary to correct in order for a patient to experience clinical benefits, Porteus says the number is likely between five and 20 percent — well below the number expected of Graphite Bio's new treatment.
»That manipulation takes all of ten minutes — zapping and adding the virus — and then we put the cell back into an incubator, let them recover for a couple days, and then they’ll be frozen away,« Porteus says.
»If it passes, then the patient will come back and we'll give them their own cells back. That transplant means the patient has to get some chemotherapy to get rid of all their own haematopoietic stem cells. We need to create space, so we use chemotherapy to get rid all of them. And then they get all their own corrected cells back through an IV transfusion. The cells can find their own way back to the bone marrow.«
»And then we will watch,« Porteus adds.
A work around or tackling the root cause?
By contrast, CTX001 — that's the sickle cell treatment developed by CRISPR Therapeutics and Vertex Pharmaceuticals — doesn't replace the mutated gene. Instead, it makes a cut that reactivates a fetal form of haemoglobin, allowing the stem cells to produce healthy blood cells through a process that lies dormant in most adults.
»The idea is to reactivate the fetal haemoglobin in beta-haemoglobinopathy patients to alleviate disease complications and improve the life quality of patients,« explains Demirci, the NIH researcher.
»In this manner, CRISPR Therapeutics is using CRISPR/Cas9 to edit a master fetal haemoglobin regulatory genomic region, BCL11A enhancer, to induce fetal haemoglobin to circumvent the severity and complications of the disease.«
So far, there's no reason to think that one strategy might be considerably more effective than the other. It's plausible that both — assuming ongoing research yields favorable results — might spur the body to produce healthy haemoglobin and alleviate the symptoms of sickle cell disease.
»In theory, both strategies are valid,« says Demirci, who adds that he's hopeful about what he's seen from both groups.
»However, we do not know yet whether these fetal globin levels are going to be stable for the long-term and how much engraftment will be obtained from corrected cells, and how much corrected cell engraftment will be enough to reverse disease phenotypes.«
More options are better for the patients
There will be a long process of clinical research for both GPH 101 and CTX001. Until then, the main difference between the two seem to stem from their methodology rather than their potential impact.
»They're turning fetal haemoglobin back on and that counteracts the effect of sickle haemoglobin,« Porteus says of the CRISPR/Vertex method.
»It's a very clever approach and it takes advantage of decades of knowledge about the disease, but it doesn't go at the root cause of the disease that I envisioned when I was a medical student, which is to directly convert the sickle gene to the non-sickle gene or haemoglobin-A gene.«
There may be slight differences between how well the two therapies end up working, Porteus says. But he drew attention to possible differences to imply that it's a good thing there may end up being multiple clinical options available, not to promote his team's approach over the other. But he also suggests that the Graphite Bio method, because it attacks the mutation directly, may end up being more generalizable to other genetic conditions than the fetal haemoglobin method. Other diseases might not have a similar indirect approach available to them, Porteus explains.
»All that said, if they both from a technical standpoint turn out to be the same, there's reason to think at a nuanced level that direct gene correction in theory should create a blood system that’s a little healthier than the fetal haemoglobin approach,« Porteus says.
»But that might come down to the assays and the measurements that the doctors argue about and the patients might not care. And there might be some difference in the patients, in how good they feel, but we just won't know until both approaches treat hundreds of patients. I think the differences might not be obvious but they will be measurable over time.«
What Porteus does think will be obvious, is that GPH101 will work much better in young kids than it does adults. That's the case for just about any gene therapy, but it's also important for sickle cell patients because the disease tends to cause more and more organ damage over time. Still, since this is a new treatment, doctors need to start by testing it out on adults — who are able to give consent to the practice — before moving on to teens and younger kids.
»We want to be able to treat this disease before all that organ damage occurs,« Porteus says. »As young as possible. But you can't start there for ethical reasons«
Need for cheap and easy option
But these are early days, and it bears repeating that Graphite Bio hasn't begun a single clinical trial on anyone, young or old. So any projection about how GPH101 will work or compare with CTX001 involves a lot of speculation and assumptions. Well-reasoned assumptions, in fairness, but assumptions nonetheless.
And even once those tests are done, assuming they go well, neither treatment will mean a thing if the people around the world who need it can't reach it.
»Although these approaches are quite promising, the processes are expensive, lengthy, and require significant expertise and [Good Manufacturing Practice] facilities,« says Demirci.
»After further optimizations, safety and efficacy assessments, we need to direct proper funding and investments and find ways to make the processes more economically feasible for successful implementation of the approaches to critical areas such as sub-Saharan Africa and India where sickle cell disease is most common and poverty is a significant problem.«
Dan Robitzski is a science journalist and former neuroscientist based in Los Angeles.
Tags
ArticleInterviewSickle Cell Disease, SCDGene therapyCRISPR-CasCas9Graphite Bio, Inc.IND - Investigational New Drug
CLINICAL TRIALS
Sponsors:
Poseida Therapeutics, Inc.
